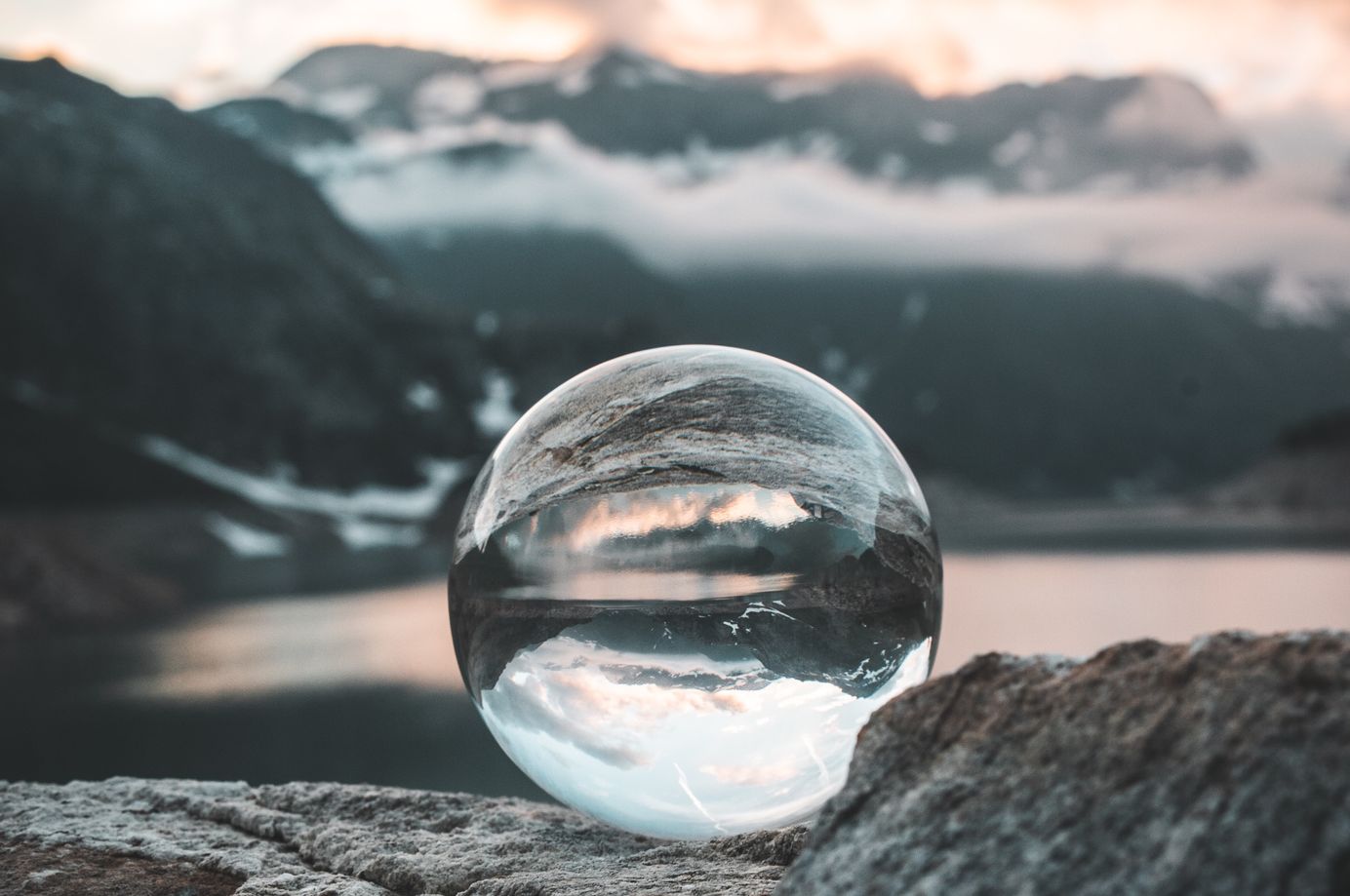
How To Get To 1 Billion Electric Vehicles Without Mining The Ocean Floor
Seabed Mining is an example of exponential technology that is destructive.

Against the backdrop of the COVID-19 lockdowns, a UN Agency and several mining companies have been making claims that to meet our commitments to climate change, there is no choice but to mine the deep ocean floor for particular metals needed for renewable technologies. These claims are pressuring many leading countries of the United Nations to take a vote in July 2020 to permit Commercial Exploitation of the Seabed.
A lot of these arguments have gone unchallenged and have been exaggerated to pressure countries to vote on a decision that will impact over half the planet’s surface in an area that scientists know very little about. This economic and resource analysis shows that mining the seabed is not necessary to meet Paris Agreement Climate commitments, and could create environmental damage at an unprecedented planetary scale if pursued irresponsibly, in what has been described as ‘history’s largest mining operation.[1]’

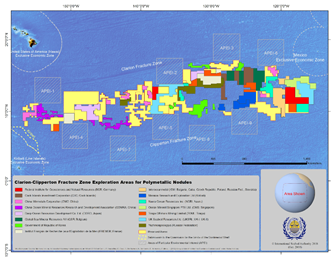
Seabed Mining Companies have been racing to persuade island nations to sign up and support their mining concession claims, such as $150m Deep Green[2] who have been backed by mining giant Glencore[3] that is 6% owned by Blackrock[4] (Blackrock’s CEO Larry Fink being famous for his public stance on environmental positions[5]). In the last few months, Deep Green has secured the support of small Pacific Island Nations Kiribati, Nauru and now Tonga for their Seabed Mining claims.
Seabed nodules in the Pacific Ocean are the first target of ocean floor mining operations, especially between Hawaii and the Californian coast - a region known as the Clarion Clipperton Zone. These nodules contain four main metals: Nickel, Cobalt, Copper and Manganese, with the focus of seabed mining operations being mainly on Nickel and Cobalt (Copper and Manganese will essentially be low-margin byproducts of the process). Nickel and Cobalt are critical parts of an electric vehicle battery, but as this economic analysis shows, there are more than sufficient resources in safe, land-based sources that have a significantly lower environmental footprint, and will meet global climate commitments.
Member states of the UN are set to vote through a UN agency called the International Seabed Authority, based in Jamaica, where 30 exploration licences[6] have already been issued to countries and companies around the world covering an area equivalent to 6% the surface of the moon, and exploitation legislation is being rushed forward for a vote in July 2020. With the world (including Jamaica[7]) under various forms of COVID-19 travel restrictions, the progress and politics of Seabed Mining Legislation will be interesting to watch in the upcoming weeks.
Claims by Seabed Mining Companies
Seabed Mining companies have made exaggerated claims to accelerate the UN vote to mine the ocean floor. Their claims have been along the following five lines:
* Scarcity: There are insufficient resources of battery materials on land to meet Paris Climate Commitments (especially Nickel, Cobalt, Manganese, Copper).
* Urgency: There is an urgency to source these materials from other locations (namely the seabed), regardless of cost, in order to meet climate objectives.
* Cost:Seabed Mining can provide materials at a lower cost than land-based mining.
* Sustainability: Seabed Mining can extract these materials with a significantly lower environmental footprint, specifically in terms of area impacted, wildlife displaced, carbon emissions, chemical reagents used.
* Tight Regulatory Oversight: These minerals can be mined from the deep ocean with sufficient international and domestic oversight to reduce any operational risks.
However, an economic and resource analysis can easily show:
* Land-based abundance: There are more than sufficient materials to meet Paris Level Agreements on land, in a way that is more sustainable than Seabed Mining.
* Major environmental risks: The risks to the earth’s system from Seabed Mining could be catastrophic, given how little we know about deep ocean ecosystems, the proposed mining techniques and potential planetary-scale impact.
* Immature Science, Regulations and Institutions: The science, regulations and institutions for Seabed Mining are not sufficiently mature to take such large decisions, and could create significant fiscal risks for smaller sponsoring states.
* No urgency: Given there is no strategic urgency to proceed with seabed mining, it would be more prudent to get the science and regulations right, rather than gamble with our planetary future pursuing an artificially aggressive timeline.
To understand the economics of the claims made by seabed mining companies, four steps need to be taken to show A) Demand for Battery Materials, B) Supply Conditions, C) Environmental and D) Regulatory Risks.
A) Climate commitments and the pathway to 1 billion vehicles
The Paris Agreement Pathway: There are three pathways to meet the Paris Agreement on vehicle emissions. First is an initial policy choice between Hydrogen Fuel Cells Technology and Electric Vehicles’ Battery Technology. Hydrogen Fuel Cell technology does not require materials from Seabed Mining, but there are other environmental implications[8]. Within Electric Vehicles, there are a range of battery technologies being explored, mainly between Lithium-Ion Batteries[9] and Solid-State Batteries[10]. Claims of lack of battery materials by Seabed Mining companies are mainly targeted at Lithium-Ion Battery technologies (rather than solid-state batteries[11]), under certain extreme edge-case conditions.
So the first step is to understand the ambition level and demand curve for electrification of the global vehicle fleet.

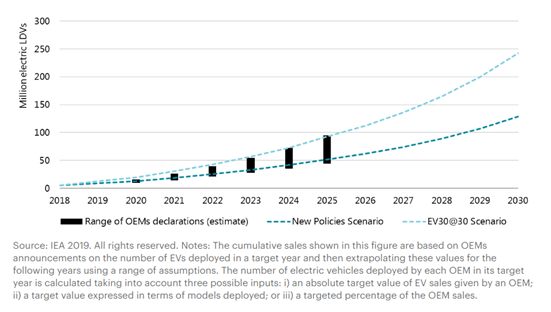
There are currently 1 billion vehicles on the road globally. The vast majority are passenger cars, with trucks, buses and two wheelers also being significant segments. All these vehicles will need to be fully replaced with electric vehicles to meet climate commitments. The key question is by when?
Ideally, there should be a pathway to reach 1 billion electric vehicles by 2040 (within 20 years), given that transportation is responsible for 24% of all man-made carbon emissions[12]. This requires replacing the existing stock of vehicles and ensuring any new vehicles are fully electric. By 2030, it is forecast that there will be 115 million new vehicles produced each year[13]. Industry estimates that between 10% and 50% of these vehicles will be fully electric by 2030, with significant variation by region (e.g., 70% of new vehicles including two/three-wheelers in China will be electric, 50% in Europe and 30% in the United States)[14].
There have been bold electric vehicle growth forecasts[15] for how aggressively the world will meet this 100% target, under various policy scenarios. International organizations such as the International Energy Agency (IEA),[16] IMF[17], OPEC[18] who continually increase their forecasts[19], consultancies and analysts such as McKinsey[20], BCG[21], Bloomberg New Energy Finance[22], investment banks such as BAML[23], UBS[24] and Morgan Stanley[25], have all produced forecasts and have broadly similar electric vehicle adoption numbers.
On electric vehicles, taking an average of all of these forecasts and scenarios the broad consensus is that by 2030, around 33 million of the 115 million new vehicles manufactured that year will be electric, with a stock of between 125 to 250 million electric vehicles already on the road by then, up from 5 million today.[26]
Industry analysts also estimate that 15% of vehicles (17 million)[27] will be fully autonomous by 2030 (which may reduce the need for the total vehicle fleet due to ride sharing technologies).


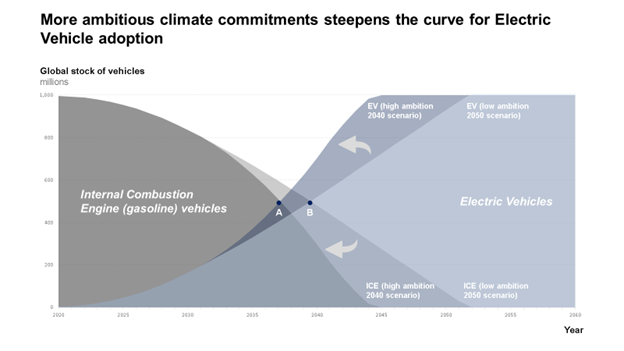


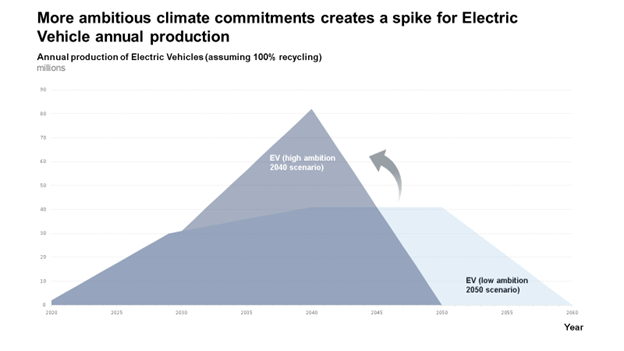
Translating electric vehicle demand into demand for battery metals
If Lithium-Ion technology is the winning technology to de-carbonize the vehicle fleet (and there are sufficiently compelling reasons to believe so), this will significantly increase demand for input materials (especially on battery technologies that displace oil and gas as the main storage of energy).

Taking a mean of the estimates and targeting 33 million electric vehicles by 2030, annual metal demand for Lithium-Ion batteries would then increase as follows:
§ Nickel demand could increase to 1.3m tons a year by 2030 (39x increase from 34,000 tons demanded annually by batteries today)
§ Cobalt demand could increase to 260,000 tons a year by 2030 (17x increase from 15,000 tons demanded annually by batteries today)
§ Manganese demand could increase to 240,000 tons a year by 2030 (21x increase from 11,000 tons demanded annually by batteries today)
§ Lithium demand (which does not come from seabed mining) could increase to 240,000 tons a year by 2030 (21x increase from 11,000 tons demanded annually by batteries today)
Supply relative to this demand will be examined later. However, it is important to understand the relative importance of these four metals in Lithium-Ion batteries:
● Nickel and Cobalt are required for the cathodes of the batteries (the part of the battery that absorbs the electrons). These two metals are the focus for Seabed Mining efforts.
● Manganese is not critical for Lithium-Ion batteries. It is used in the cathodes of batteries, but only as a stabilizing material[28]. It is one of the most common elements on Earth (the 12th most abundant material [29]in the earth’s crust), so supply is not a concern. With demand for manganese critical for other industries (such as stainless steel[30]), land-based production is likely to continue at robust levels. Due to its abundance and low cost, most electric vehicle companies use Manganese in their batteries as a stabilizing material (Nickel, Manganese, Cobalt or NMC batteries). However, with recent battery technology innovation, Manganese is even being replaced by Aluminium as the stabilizing material, with Tesla opting for Aluminium in its batteries (Nickel, Cobalt, Aluminium or NCA batteries). Either way, there are more than sufficient resources on land for Manganese and Aluminium, so these metals are less important as a rationale for Seabed Mining.
● Lithium is not being sought from Seabed Mining sources. Lithium can also be easily replaced at a later stage with less environmentally damaging alternatives like Silicon with advances in new battery technologies[31].
Even with these forecasts, there are two dampening forces that are likely to reduce the intensity (and amount) of metals needed for electric vehicles over the next few years. Improved battery chemistry and greater recycling. Let’s take each in turn.
Improved Battery Chemistry could reduce Cobalt demand by 85%

Within Lithium Ion battery technologies, there are several important technological advancements that improve the performance of electric vehicle batteries and affect the ratios of Nickel, Cobalt and Manganese in each battery.
Current trends are moving from NCM 111 to NCM 622 to NCM 811, implying moving from ratios of 1 part Nickel, 1 part Cobalt and 1 part Manganese[32] (33% of the battery being Cobalt), to 8 part Nickel, 1 part Cobalt, 1 part Manganese (10% of the battery being Cobalt).
Some forecasts go even further to 9 parts Nickel, 0.5 parts Cobalt and 0.5 parts Manganese (5% of the battery being Cobalt). Hence, there is likely to be significantly less Cobalt needed for Electric Vehicle batteries in the future[33](more than 85% reduction in Cobalt per battery from today).
Tesla has gone even further and has been exploring Lithium-Ion Phosphate Batteries (LFP) for certain (mainly China) markets, that remove the need for Cobalt altogether[34]. So the original Cobalt demand figures are likely to be at the higher end of any estimates.
Battery Recycling Technology is accelerating due to public and regulatory pressure.
At present, about 50% of metals from electric vehicle batteries are recycled. The main reason is due to the manufacturing process, which has made it difficult and costly to extract the metals from batteries for recycling. This is also the reason why consumer electronics have lower battery-metals recycling rates (due to their small size and complex assembly).
However, regulatory and public pressure has driven battery manufacturing innovation toward more simplified assembly processes. Current forecasts are for electric vehicle battery recycling rates to increase to 75% by 2030[35].
So once a finite amount of resources have been mined to meet the demand of 1 billion Electric Vehicles, these materials should largely remain in a steady state of reuse at the end of life for each vehicle[36]. As the rate of innovation in recycling technologies increase, this is likely to result in fewer virgin metals needed to be mined for electric vehicle batteries, and should continue to be a focus for policymakers and innovators.
Hence with both improved battery chemistry and greater recycling rates, the world is likely to need significantly lower amounts of the metals to meet Paris Agreement Commitments.
Pricing implications for electric vehicles
So far, the analysis has just explored the amount of material required to meet annual demand of 33 million electric vehicles by 2030 (and a stock of 200 million vehicles of the total 1 billion fleet).
It is equally important to understand the pricing sensitivity given availability of battery materials to see the overall impact on affordability and competitiveness of electric vehicles in the market.
Industry Analysts not concerned about global supply
Pricing is often an indicator of how mining analysts view abundance or shortages of certain commodities in the market. Price spikes are often indicators of likely shortages, and lower prices indicative of an oversupply in the market. With Nickel and Cobalt, Commodities Futures Markets do not appear to be concerned with the ability of the market to meet future demand scenarios.
Since the end of the commodity super-cycle boom in 2008, prices of both Nickel and Cobalt have fallen in line with their historic lows, even with the discussion about rapid adoption of Electric Vehicles. Hence industry analysts and the market have priced in demand and supply, and do not see a supply pinch over the next few years. Mining Industry Analyst reports often cite that there are sufficient supplies of both minerals in existing land-based mines, warehouses, and with many low-cost and low-polluting new sources coming online in the next few years, that can easily be shown by looking at global supply forecasts.

Nickel prices have not increased much more than the price of Cheese (with a spike during the end of the commodity super cycle in 2008)
Impact on Electric Vehicle Prices


For context, batteries currently account for one third the cost of an average electric vehicle, and are falling in cost by over 20% each year (see charts above). One of the main drivers for the fall has been manufacturing process innovation that has improved the ease with which Lithium-Ion batteries can be incorporated into existing car production facilities, as well as falling costs due to at-scale Lithium-Ion battery recycling processes.
So, whilst alternative technologies like solid state batteries may have more favorable properties (e.g., more rapid charging profiles), it may be challenging for these technologies to be incorporated in large scale vehicle assembly plants over the next decade. So Lithium-Ion batteries are likely to be the quickest path to get to 1 billion vehicles over the next 20 years, and at increasingly affordable rates due to economies of scale.
What are the pricing sensitivity implications of various raw material scenarios?
Today, the price of a battery for an average family-size $45,000 electric vehicle costs one third, or $15,000. The overall cost of a battery is forecast to almost half to $8,000 within 10 years due to improved battery manufacturing[37]. About half ($4,000) of this is due to raw materials (i.e., Nickel, Cobalt, Manganese). Based on a battery chemistry of NCM111, each metal accounts for one third of this raw material cost (so $1,333 for Nickel, Cobalt and Manganese). So for an electric vehicle costing $45,000, less than 10% is battery raw material cost, and less than 3% are the costs of Nickel, Cobalt or Manganese.
Bloomberg New Energy Finance did a sensitivity analysis with the more advanced NCM811 battery chemistry. It shows that even if prices of Nickel were to double (i.e. to $24,000 a ton, as shown by the blue bubbles in the chart), this will only lead to an increase of 5% in the overall vehicle battery cost and 2.5% increase in the overall price of an average electric vehicle. This is a negligible risk to meeting the 1 billion electric vehicle target, compared to the unknown risks of large scale commercial seabed mining.

Each metal has their own market dynamics and supply considerations. It’s important to understand each in turn, starting with the commodities that are highlighted as most important by Seabed Mining Companies.
B) Supply Conditions
1. Nickel
Annual global battery demand for Nickel is likely to be 1.3 million tons by 2030. Today, annual production is 1.2 million tons. However, it is important to understand the likely evolution of land-based supply - i.e., it is not one of mineral shortage – and the higher quality of Nickel found on land compared with on the ocean floor.

The underground Nickel sulfide vs surface Nickel laterite story
Seabed Mining companies have made claims that land-based Nickel mines are low grade, high cost and environmentally destructive. However, there are two categories of Nickel Mines that are important to distinguish.
Land-based Nickel occurs naturally in the earth’s mantle and is geologically formed from magma intrusions into the Earth’s crust. This results in Nickel resources being divided into two categories, based on how far the magma protrudes into the crust: sulfides or laterites[38].
Nickel Sulfides are the result of being produced in large magma chambers that do not penetrate the Earth’s surface. They are then chemically refined[39] over millions of years in large magma chambers just below the surface[40]. These are extremely attractive, as Nickel Sulfides contain very high grade metal ore (between 2.2% - 4.0%), are very concentrated along seams in the Earth’s crust (reducing the environmental footprint of mines), and require less waste, less energy-intensive processing, and less complex chemical separation. They are typically mined underground.
Nickel Laterites occurs when magma has reached the surface and is chemically weathered down and diluted over millions of years, just below the soil surface. This results in significantly lower grade material (at 1.3%, this is half the quality of Sulfide deposits) that is rarely mined. So whilst Nickel Laterites make up 70% of the world’s nickel reserves, it has been Nickel Sulfides that have produced 60% of the world’s nickel over the past 60 years[41]. Where Nickel Laterites are mined, this results in large, destructive, open cut surface mines[42] that require significant chemical and energy-intensive processing to separate the low-grade Nickel (a process known as High-Pressure Leaching or HPAL, which also requires sulfuric acid)[43]. This results in large, toxic ‘tailings’ or waste materials from the mine[44]. Typically Nickel Laterites from this oxidation process are usually found in tropical and subtropical regions near the equator.
In short:
Nickel Sulfides
§ More concentrated (less destructive) underground mines
§ Higher Grade (60% of Nickel has been mined from Nickel Sulfide sources). Grades typically between 2.2% and 4.0% in the most productive mines.
§ Lower cost due to concentration
§ Less energy intensive and fewer chemical reagents
§ Many major new discoveries coming online to meet growing demand
Nickel Laterites
§ More expansive and destructive open-cut, surface mines
§ More abundant (70% of global nickel reserves are laterites)
§ Lower Grade (typically 1.3%)
§ Higher cost (though at $15,000/ton, there is abundant supply of Nickel Laterites)
§ Higher energy intensity
§ Higher reagents and toxic chemical tailings
We will soon come to see that Nickel from Seabed Mining resembles the profile of Nickel Laterites, that is:
Nickel Nodules from Seabed
§ More extensive destruction to seabed (similar profile to surface-based Nickel Laterite Strip Mining rather than the concentrated underground Nickel Sulfide mines)
§ Lower Grade (typically 1.3%, which is similar to surface Nickel Laterite mines)
§ Higher energy intensity and higher amounts of chemical reagents needed to separate the Lower Grade Nickel Ore
§ Current costs do not accurately reflect the environmental damage or royalty regime (hence exaggerated claims that seabed mining for Nickel is at the lower part of the industry cost curve)
§ Significance in how these nodules were formed – produced biologically over millions of years from microscopic microbes extracting trace elements from seawater, rather than geologically through magma.
Nickel Industry Cost Curve

The Nickel Supply Curve (made up of the production cost breakdown of every Nickel mine) reveals how attractive Nickel Sulfides are, and also that at a certain price point (around $15,000 a ton), there is an almost limitless supply of Nickel Laterites (i.e., there is no global shortage of Nickel that requires Seabed Mining to meet Paris Agreement Commitments).
This is easy to see on the Nickel Supply Cost Curve. High-grade underground Nickel Sulfides supplies are marked by large production volumes and lower cost (mainly to the left of the cost-curve with a few giant mines dominating production, such as the large Nova-Bollinger Mine in Australia). The lower-grade surface Nickel Laterite deposits can be identified by relatively high costs of chemical reagents, more energy intensive processes and other onsite costs such as managing toxic tailings (colored bright green, dark red and purple respectively). This makes up over half the costs per ton of Nickel produced. As a result, this pushes Nickel Laterite Mines to the right (more expensive) side of the cost curve. This is a similar cost and production profile to what we expect to see from Seabed Mining for Nickel Nodules.
Before we turn to claims made by the Seabed Mining Industry, it is important to understand how land-based mining supply is likely to evolve.
Best case scenario for Nickel: Huge New Nickel Sulfide Discoveries
Very little of the earth’s crust has truly been explored. Historically, only the top 100m of the surface has been mapped using traditional techniques from over 50 years ago. This means less than 10% of the earth's surface has been mapped geologically in any meaningful way (below 100m). Using new drone and satellite technologies as well as machine learning capabilities, geologists, analysts and engineers are able to see further into the earth’s crust (beyond 100m depth) and have developed advanced technologies to better assess mineral resources. This has resulted in new discoveries of massive underground Nickel Sulfide deposits in recent years that are likely to come online within the next decade if prices were to rise slightly.
Contrary to claims made by the Seabed Mining Industry, some of the best new sources of Nickel Sulfides have only been discovered in the past 15 years, and have rapidly come online within 5 years of discovery. This implies many more major discoveries are imminent and can rapidly be brought online. For example, the massive underground Nova Bollinger Nickel-Copper Mine[45]in Fraser Range[46], Western Australia was only discovered in 2012, and came online within 3 years in 2015. Today, it is one of the world’s largest, lowest cost and highest grade Nickel mines, as seen on the left of the industry supply curve[47]. It produces enough Nickel each year for almost 2 million electric vehicles batteries[48]. Promising new deposits such as the Ntaka Hill[49] Nickel Sulfide mine in Tanzania discovered in 2006 with a mineral resource of 1.8 million tons[50]and expansion projects such as Garibaldi’s Nickel Mountain in the Canadian Province of British Columbia show that we have barely scraped the crustal surface of known and unknown Nickel land deposits that are large, accessible, economically viable and sustainable[51]. These are just some of several strong underground Nickel Sulfide prospects coming online enabled by new technologies[52].
Worst case scenario for Nickel: Nickel Laterites to meet demand
There is a three step prioritization to source Nickel. First, source from underground Nickel Sulfides (where the highest quality resources cost $9000 a ton). Then from surface Nickel Laterites (where the grade is lower, but there is almost a limitless supply at $15,000 a ton). Only then would Nickel from Seabed Mining make economic sense if all land-based supply had been exhausted and if the seabed nodules had a materially higher ore grade quality.
In the unlikely scenario that new underground Nickel Sulfide discoveries do not materialize or rapidly come online, there are more than sufficient Nickel Laterites resources to meet Electric Vehicle demand without the need to mine the Seabed. There are billions of tons of Nickel Laterite reserves around the world, which is more than sufficient to meet Electric Vehicle demand of 1.3 million tons a year by 2030[53]. Even if prices were to increase 70% to $15,000 a ton, this would only result in a 5% increase in the price of an EV battery (2.5% price increase to the overall electric vehicle). And this is for land-based mines where the risks are known and can be managed for a slightly higher price (that will not disrupt pricing or supply in the electric vehicle market).
Whilst speculators have pushed for a narrative of global shortages of Nickel and Seabed Mining companies have made exaggerated claims that Seabed Nodule Nickel costs will be at the lower end of the supply curve, experienced market analysts have not seen the need to push prices higher than their historic lows (even before the COVID-19 pandemic) of $12,000 a ton. This reflects the consensus and judgement of experienced market analysts that there is unlikely to be a Nickel shortage in the near future.
Seabed Mining has a similar profile to Open Cast Nickel Laterite Mining
Seabed Mining companies have claimed that Nickel from nodules are ‘high grade.’ The grade of the ore has to be published with the International Seabed Authority and is publicly available. However, an analysis of published results show that Seabed Mining company claims of High Grade resources are not the case. Seabed Nodules resources are more in line with open-cast Nickel Laterite Mines.
§ Underground Nickel Sulfide average ore grade: 2.2% - 4.0% (S&P Global on Nova-Bollinger)
§ Surface Nickel Laterites average ore grade: 0.5% - 1.3% (S&P Averages)
§ Seabed Mining nodules grade: 1.3% (ISA Reports and Mining Company Disclosures)
So on average, the main land-based Nickel mines – primarily, Nickel Sulfide mines – is up to300% Higher Ore Grade than the average 1.3% Nickel ore grades found in Seabed Nodules, which is classed as Low-Medium Grade, in line with open cast Nickel Laterite Mines.
This implies a chemical separation process (e.g., HPAL) of Nickel from Seabed Nodules, requiring sulfuric acid and disposal of toxic tailings. A like-for-like comparison is therefore needed for the full impact of Seabed Mining, that should also include any transport, processing and separation of the Nickel from the polymetallic seabed nodules.

Verdict on Nickel: In summary on Nickel, there is no need to mine the Seabed for Nickel. Annual demand for EV batteries is forecast to increase to 1.3m tons by 2030, from today’s annual supply of 1.2m tons. Large deposits of High-Grade Nickel Sulfide Mines are likely to come online in the next few years at $9,000 a ton. In the unlikely event that situation does not occur, there is almost a limitless supply of Nickel Sulfides at $15,000 a ton. These will be at a similar ore grade (Low-Medium), price, and abundance of minerals as found on the Seabed. However, the environmental risks of land-based Nickel Laterite Mines are known and understood, compared with significant unknown environmental risks of inferior Nickel from Seabed Mining, where more time could allow the effects to be fully studied and understood.
2. Cobalt

The other metal upon which the Economic case for Seabed Mining has been made is Cobalt. Earlier, we showed that at the top end of estimates to meet demand for 1 billion Electric Vehicles, Cobalt supply for batteries needs to increase by 17x from 15,000 tons a year today to 240,000 tons by 2030. Over the next few years, there is more than sufficient resources in existing mines for production to increase 6-fold, or by over 100,000 tons a year. Over the next twenty years, annual global production then needs to double from 115,000 tons a year to 240,000 tons, implying new discoveries will need to be made (assuming current rates of Cobalt consumption in existing battery technologies). The challenge in the short term is not supply or availability of Cobalt, but where most of the world’s current mines and 68% of current supply is located[54]: the Democratic Republic of Congo and the associated geopolitical risk.
Cobalt is not in fact a rare metal. It almost always exists as a byproduct of Copper or Nickel mining, hence the economics of Cobalt is strongly linked to the associated metal ore. The Katanga and Lualaba Provinces in South East DR Congo have some of the highest-grade resources of Copper and Cobalt in the world[55]. As the copper mines were developed, this helped reduce the cost of developing Cobalt resources. 90% of the value of these mines were therefore from Copper and 10% from Cobalt, hence the focus was on optimizing for Copper exploration and production. This means relatively little effort was spent on exploring for high grade Cobalt in the past. Due to the high ore grade in the DR Congo, today over 68% of global Cobalt production is mined from this region[56]. Three giant mines there currently dominate over 50% of the global Cobalt supply, as can be seen from the large supply blocks in the industry supply curve: Glencore owned Mutanda Mine[57] and Kamoto Mine[58], as well as China Molybdenum owned Tenke Fungurume Mine[59]. Lower cost Cobalt is also available from a series of smaller, artisanal mines in the DR Congo, but these carry considerable risk. Several incidents over the past five years highlighted the instability and challenges of operating in the DR Congo and sent shockwaves through the Cobalt market, driving prices up 300% (before a subsequent normalizing) and triggering a series of events that will soon diversify supply away from the DR Congo, and reduce the need to mine the seabed for Cobalt.
On the geopolitical risk side, President Joseph Kabila was due to step down in 2016 after serving two Presidential terms and being in power since 2001, but elections were delayed until 2018. This triggered months of political unrest and protests across the country that rocked 68% of the global Cobalt supply chain. The Government then enacted a new Mining Code[60]that made Cobalt a ‘strategic mineral’ and tripled Cobalt royalty rates to 10%,[61]leading to strong objections from the mining companies and raising the prospect of resource nationalism[62]. At the same time, allegations of corruption and bribery were made against some of the biggest Cobalt miners in the world,[63]which eventually led to major US Department of Justice and UK Serious Fraud Office investigations into Glencore’s operations in the DR Congo[64]that could have wide reaching consequences. Over this period, Amnesty International released a high profile report[65]highlighting child and labor abuses in the artisanal mining Cobalt supply chain, ultimately linking the supply to 24 global technology and electric vehicle customers[66]. This eventually triggered a series of lawsuits against these major technology companies,[67]highlighting further risk of sourcing from poorly regulated artisanal mines in the DR Congo. Significant efforts, focus and resources have subsequently gone into addressing these challenges within the DR Congo, including the January 2020 creation of a State Purchasing Monopoly (a state-backed Gecamines subsidiary) on Cobalt produced from Artisanal Mines for the next five years, with strong provisions against child labor[68].

Against the backdrop of initial hype around growing demand for electric vehicles, geopolitical risk in the DR Congo and a potential shortage of cobalt supply, speculators rushed into the market, driving prices up 300% between 2016-18. However, this spurred new investments into Cobalt production that rapidly came online and led to a subsequent 70% price fall[69]. There was so much new supply of Cobalt, that major mines reduced capacity, with Glencore announcing plans in September 2019 to temporarily close the giant Mutanda Mine[70] due to oversupply in the market and subsequent low Cobalt prices[71]. Mutanda Mine is responsible for 20% of all Cobalt production in the world and is currently on ‘care and maintenance’ until demand picks up again[72].
A wave of major new High Grade Cobalt discoveries
As a result of the perceived risk to Cobalt supply posed by DR Congo operations, substantial new investments have begun for Cobalt in existing and alternative locations. Revolutionary new data science, machine learning and satellite technologies are identifying substantial, high grade resources outside of the DR Congo,[73]in safe and stable regions that were previously unexplored (remember, less than 10% of the Earth’s land crust area has been meaningfully, geologically explored). Several recent discoveries have been extensions of current projects in Canada[74], Russia[75]and Finland[76], which is not a small feat. It is difficult to find Seabed Nodules of Cobalt Ore even close to the quality and volume of currently discovered land-based deposits. The largest producers of cobalt in order are DR Congo, Russia, Australia, Philippines, Cuba, and Madagascar.
To increase supply even further, in 2019 the United States declared Cobalt a ‘critical mineral’ and together with nine countries have increased resources to identify and develop new Cobalt resources[77]in strategic locations. Many promising, High Grade (above 3.0% grade) finds around the world are emerging in countries such as Australia[78], Canada[79], Kazakhstan[80]and the USA[81]and have triggered a race to bring these substantial new Cobalt resources online to meet projected demand for electric vehicles.
It is clear that there is not likely to be a substantial shortage of High-Grade Cobalt from safe, geopolitically stable, land-based sources to meet Paris Agreement Climate commitments and that these resources can be brought online rapidly and safely. These market dynamics reflect themselves in the historic low current spot and futures prices of Cobalt.
Land-based Cobalt is 1500% Higher Grade than Seabed Nodules
With Cobalt, new land-based sources are being discovered at 3.0% grade and above[82]. This is 1500% greater than the average 0.2% Cobalt grade found in Seabed Nodules in the Clarion Clipperton Zone.
Extracting Cobalt from Seabed Nodules is 126% more carbon intensive
In a White Paper put out by the Seabed Mining Industry, it is even shown that extracting Cobalt from the Seabed is 126% more Carbon intensive than from land-based sources (page 107[83]). This was arrived at using an Economic Allocation Weighting for the Cumulative Energy Demand Model that was used for an industry self-published Cobalt Life Cycle Analysis.
Decline of Cobalt in EV batteries

Even in an unlikely worst case scenario if Cobalt Prices were to double as demand moves to the right hand side of the supply cost curve or higher standards in the DR Congo result in higher prices, this would only lead to a 3% increase in electric vehicle battery price, following a sensitivity analysis conducted by Bloomberg New Energy Finance, shown in the earlier chart[84]. Again, not a major disruption to the pathway to 1 billion vehicles and relatively lower risk than Seabed Mining.
Cobalt is also likely to fall in relative importance as battery technology developments reduce the amount of Cobalt required per battery as batteries move from NCM111 to NCM811 (and potentially even halving to 0.5 Cobalt), in the rush to meet 33 million vehicle annual demand by 2030. Indeed, Tesla CEO Elon Musk made a commitment in 2018 to reduce Cobalt consumption to ‘almost nothing’ and was recently said to be in discussions with major electric vehicle battery producer CATL around technologies that would not use Cobalt at all[85].
Cobalt Verdict: All in all, there is likely to be sufficient Cobalt supply from land-based sources and in stable locations outside of the DR Congo within the next decade to meet the forecast demand for electric vehicles without any major disruptions, and without the need to mine the Seabed for inferior Cobalt that has significantly (15x) lower grade and emits 126% greater concentration of carbon per dollar of Cobalt.
3. Manganese

Manganese is the 12th most abundant element in the Earth’s crust. It is not a critical material for electric vehicle batteries (as a stabilizing material in the Cathode), and can also easily be replaced with Aluminium as Tesla is doing. To meet demand for 33 million vehicles by 2030, 240,000 tons of Manganese a year will be needed for batteries. Access to Manganese should not be a bottleneck as this is a fraction of today’s production and global reserves.
Almost 19 million tons of Manganese are mined a year, with South Africa being the biggest producer responsible for one third of global production at 6 million tons a year.[86]This industry generates over $3 billion a year for the South African economy[87], creates over 10,000 jobs and is growing 10% a year with new infrastructure developments[88].
50% Higher Manganese Ore Grade on Land vs Seabed Nodules
South Africa, Australia, Brazil and Gabon all have the highest reserves of High-Grade Manganese (over 44% Ore Grade, which is 50% higher than the average 29% Manganese Ore Grade with Seabed Mining Nodules[89]), with South Africa alone having over 200 million tons of High-Grade reserves[90]. Manganese prices remain at multi-year lows, reflecting oversupply in the market[91].
63% Higher Carbon intensity from Seabed Mining Sources
Indeed, even a Seabed Mining industry assessment[92]of extracting Manganese from Seabed Nodules shows that the Seabed Mining process would emit 63% higher Carbon Dioxide emissions per ton of Manganese extracted compared with land-based mining.
This was again using the industry-self published Life Cycle Analysis and Cumulative Energy Demand Models using Mass-Based Allocation methodology (page 107[93]).
Verdict on Manganese: Hence mining the seabed to meet Manganese demand for electric vehicles is not justified, with no production bottlenecks anticipated over the next decade, and Seabed Mining for inferior Manganese shown to be 35% lower grade and 63% more Carbon intensive than abundant land-based resources.
4. Copper

Seabed Mining companies recognize that copper will be a by-product of seabed mining operations of polymetallic nodules, and estimate that copper will account for only 1% of the metal extracted, so have tried to identify copper as strategic to meeting Electric Vehicle demand. However, looking at land-based industry demand, seabed mining for copper is not justified.
With copper, only 12% of known global reserves of 5.8 trillion tons have been mined, and nearly all is still in circulation due to copper’s high recycling and performance rates. Indeed, in the US, almost as much copper is recovered from recycled ore as is mined from the ground[94]. Today’s global mining production is 21 million tons a year[95], with Chile being the largest producer at almost 6 million tons a year[96]. Forecasts are that demand for copper due solely to electric vehicles will be 1.6 million tons by 2035[97], a fraction of today’s global production and reserves. This is reflected in low and declining copper prices[98], with plenty of prospects for high grade land-based copper resources if demand picks up[99].
50% higher Copper Ore grade in the largest, most attractive land-based mines
The Seabed Mining industry claim that land-based Copper mines have seen declining ore grades over the past decade. This is more a reflection of lower investments in copper mines, not resource depletion. Many new Higher-Grade copper mines like the massive Oyu Tolgoi copper mine in Mongolia are being developed that have the potential to be 13% of global production with ore grade seams of 1.7% (and as high as between 2% to 5% in parts)[100]. This is on average 50% higher than average Copper Ore Grades from Seabed Nodules of 1.1% from the Clarion Clipperton Zone. The end of the copper super cycle resulted in slow down of investments into copper mining, not necessarily depletion of resource or lower quality.
Verdict on Copper:Access to copper will not be a bottleneck to achieving electric vehicle climate commitments, many large, high quality resources exist on land that are 50% Higher Ore Grade than from Seabed Mining, so Seabed Mining for copper alone is not justified.
C) Pricing in Environmental Risk
The Seabed Mining Industry relies on six main environmental arguments to justify why Seabed Mining not only addresses strategic mineral shortages (that we were unable to identify) but also why it is more sustainable than land-based mining. In an industry White Paper[101], the industry claims that compared to land-based mining, Seabed Mining will result in:
§ 70% less CO2e direct emissions
§ 94% less stored carbon at risk
§ 90% reduction in SOx and NOx emissions
§ 100% reduction in solid waste
§ 94% less land use
§ 93% less wildlife at risk
It is clear that these are exaggerated claims using selected statistics (e.g., comparisons with low-grade, open-cast Nickel Laterite Mines rather than high-grade underground Nickel Sulfide Mines), and not like-for-like comparisons (e.g., ignoring mineral processing and sediment plumes from seabed operations) which distorts and misrepresents the true environmental risks of Seabed Mining.
Land-based mining is not without its well-publicized environmental, social and regulatory challenges,[102]especially Cobalt Mining in the DR Congo and Nickel Laterite open-cast mines in Asia[103]. However, there is growing momentum along the supply chain to address these issues rather than blindly risk the environmental liabilities of opening up a new frontier with unknown, high-risk consequences in the ocean. It is important to compare and mitigate the relatively understood environmental risks of land-based mining rather than chance the highly uncertain ‘unknown-unknown’ risks of Commercial and Industrial-Scale Seabed Mining. Let’s look at each in turn.
Environmental reforms within land-based mining
The media, civil society organizations, end customers and Governments are increasing their scrutiny over social, environmental and regulatory issues in land-based mining in the years to come as consumer electronics and electric vehicles increase in market share and importance. So far, there have been several important steps that actors across the supply chain have taken.
Through advice from the OECD[104], the London Metals Exchange[105](the world’s largest trading marketplace for industrial minerals) has been making efforts to ensure full traceability and sustainability of minerals that are traded on their exchanges[106]through a Responsible Supply Chain Initiative[107]. These are well established practices and was used as part of the Kimberley Process[108] to prevent Conflict Diamonds from entering global supply chains as was highlighted in the Leonardo DiCaprio movie, Blood Diamond[109].
Efforts to improve transparency, traceability and sustainability along the supply chain are likely to continue as consumer electronics and electric vehicle companies make stronger commitments for safe, clean and green supply chains, in the face of corruption allegations against the large Cobalt miners in the DR Congo[110]and child labor abuses among the artisanal mining sector. As production and new sources of Cobalt are developed outside of the DR Congo, it will be possible to achieve such sustainability and social objectives without a shortage or large price spikes of Cobalt or electric vehicle batteries (as highlighted earlier).
Even the DR Congo Government has recognized the gravity of the situation and in January 2020 introduced strong and draconian measures to strengthen their control of the market and regulate artisanal Cobalt mining to ensure no child labor occurs along the supply chain[111].
On Greenhouse Gas Emissions from Mining Operations (which account for 7% of total Greenhouse Gas Emissions[112]), many of the major mining companies have made strong commitments to reduce emissions[113]and are rapidly deploying renewable technologies to mine sites around the world.[114] This is particularly so in remote and sunnier locations in Australia and parts of Africa where solar can be set up more easily and heavy fuels are more difficult to transport. These are also the locations of the world’s largest Nickel and Cobalt mines.
Clearly, the move toward greater use of renewable technologies to power mines needs to be pursued more ambitiously if Mining Operations are to remain within Paris Climate Commitments, and when the UNFCCC Paris Accord comes into force sometime in the next twelve months[115], greater momentum may be seen here.
Pricing Unknown Ocean Ecological Risk
In comparison, there are several major environmental risks with Seabed Mining that have not been priced into the claimed costs or environmental insurance for Seabed Mining. Here are six of the most important. Several are ‘known-known’ risks, others are ‘known-unknown’ risks, and even more concerning are the ‘unknown-unknown’ risks. In order of progression of risk:
§ Carbon Intensity: Compared with land-based mining, Seabed Mining Industry’s own figures show Seabed Mining for Manganese is 63% more carbon intensive and Cobalt 125% more carbon intensive than land-based mines. These figures could be underestimates and should be open to International Expert Peer Review in respected and well-established Scientific Journals, before any more public claims are made by the Seabed Mining Industry.
§ Low Seabed Mining Grades and more Chemical Reagents: Taking the published data on Clarion Clipperton Zone Nodules and comparing this with land-based sources, we see that in every case Ores from the Seabed are significantly lower than average land-based prospects.
o Nickel: Seabed Nodules 1.3% Grade vs Land-Mines 2.2%-4.0% Grade (as much as 300% higher than Seabed Mining)
o Cobalt: Seabed Nodules 0.2% Grade vs Land-Mines 3.9% Grade (as much as 1500% higher than Seabed Mining)
o Manganese: Seabed Nodules 29% Grade vs Land-Mines 44% Grade (as much as 50% higher than Seabed Mining)
o Copper: Seabed Nodules 1.1% Grade vs Land-Mines 1.7% Grade (as much as 55% higher than Seabed Mining)
Lower grade minerals imply a greater amount of energy and chemical reagents will be required for separation of Seabed Nodule metals (similar to the Nickel Laterite Open Cast Mining models). This is a non-trivial process and should be open to expert scrutiny to understand where this environmental risk is being displaced to (e.g., the sediment plume or coastal or island operations close to Seabed Mining Operations that create new toxic waste), prior to any regulations being approved. This is a ‘known-known’ risk and requires full operational transparency.

§ Noise, Light Pollution and Habitat Destruction: The deep ocean habitat has evolved since before humans walked the Earth. The processes for life at the deep ocean floor are radically different to that on the surface that relies on photosynthesis, and which science only discovered in 1977. The microbes and lifeforms that inhabit the deep ocean environment have evolved over tens of millions of years in the dark, without noise or light pollution. Noise travels much further in the deep ocean – often thousands of miles,[116] and can be disruptive to wildlife not used to artificial sound. Seabed Mining operations will entail large scale ‘combine-harvester’ size machines operating noisily at the Seabed Bed semi-autonomously, and requiring light to monitor operations.
It is critical to understand the implications of introducing such disruptions to these pristine environments, as well as displacing the solid nodule upon which many species depend to breed and feed. In order to meet public production schedules across various mine sites, the scale of multiple operations is likely to be very significant across the Pacific Ocean and Indian Ocean. Scientists do not yet fully understand these deep ocean habitats and what marine life lives there. Such noise, light and habitat destruction is dangerous and can destabilize this ill-understood habitat. This is the reason why there is a commercial mining moratorium in Antarctica until 2048 signed by 45 nations through the Madrid Protocol in 1991,[117] and a similar 16 year Commercial Fisheries Moratorium in the Arctic signed by the EU and the 9 major Arctic Fishing Nations in 2017 as the impacts of climate change on life there are studied and better understood[118]. These are all modelled after 1982 moratorium on commercial whaling that now has 88 country signatories[119].
Humans only just discovered life around deep-ocean hydrothermal vents forty years ago[120] and much of the area around hydrothermal vents in the Indian and Atlantic Oceans are being licensed for Seabed Mining[121]. Since life was discovered in 1977, over 700 new species have been discovered, almost two a month[122]. These are the ‘known-unknown’ risks, that require a more robust, international peer-reviewed Environmental Impact Reporting standards than are currently in place to understand the parameters of the risk.
§ Wildlife disrupted: Some preliminary studies have revealed what life exists around the Seabed. Already, a newly discovered species of deep ocean Octopod now known as ‘Casper’[123](named after its ghostly appearance) was revealed to only deposit its eggs on seabed nodules, and would become critically endangered if these nodules were to be removed from large parts of the Pacific Ocean[124]. Microscopic microbes at the bottom of the ocean have been around longer than humans and evolve at geological timescales. It is believed some of these microbes are the ones that have produced the nodules in the first place from trace elements in the water, and grow at a rate of 1 millimeter every 1000 years (so these ecosystems will never recover within human timescales again). This is deeply profound, implying Seabed Nodules are biologically produced, which is a radically different process of magma-based intrusions into the Earth’s Crust for land-based mining. Colonies of such enzymes vary significantly from nodule to nodule, hence it is critical that these microbes are studied and understood, especially any role they play in deep ocean carbon and nutrient cycle. Indeed, if it is shown that these microbes are the ones that have biologically produced the nodules, this in itself could be more valuable than the mineral properties. These are ‘known-unknown’ risks based on deep ocean wildlife already identified. Indeed, where experimental seabed mining was conducted off the coast of Peru in the 1980s, over 70% of visible life had not returned over 25 years later[125]. This shows the impact a quarter of a century later based on what technologies existed then to take species baselines[126].
§ Novel Organisms:Beyond species already identified, there are likely to be significantly more species, currently unknown to science, and whose role in deep ocean ecosystems are yet to be understood. These are the very processes from which life on this planet could have originated and which we know so little about. Less than 0.05% of the deep ocean floor – an area the size of Tasmania - has been visited, photographed, videoed or mapped using sonar at less than 5km resolution[127]. The latest environmental surveys should entail eDNA sampling and microbial assessments to get a sense for how many species are unknown to science in the deep ocean around Seabed Mining Concession Zones. These unknown organisms could be critical for various applications such as bioremediation, industrial, agricultural and medical purposes. Indeed, over forty enzymes from the deep ocean have already been identified as potentially having applications against Coronaviruses[128]. These are the ‘unknown-unknown’ environmental risks which makes Seabed Mining a radically different proposition from how land-based mining is being evaluated, and where a pathway to resolve these information gaps are essential before any Commercial Mining Concessions are agreed upon. The Seabed Mining Industry claims that there is 93% less wildlife at risk from ocean operations is fallacious at best.
§ Sediment Plumes: Perhaps the biggest risk of all, that is also an ‘unknown-unknown’ risk, is that of sediment plumes[129]. These are the sediments from the ocean floor that are displaced when Seabed Mining operations begin and nodules are extracted (for Seabed Nodule mining, compared with Hydrothermal Vent Mining). This will be released in environments that are very poorly understood and which have been undisturbed by humans (especially at over four miles depth). There are several strata of slow-moving currents and habitats that have not seen noise, light or sediment for millions of years. Microscopic organisms have evolved in these very specific environments and are unique to the planet and critical to several earth processes.
There are several degrees of uncertainty around sediment plumes, such as the amount of sediment that will be displaced under different mining technologies (e.g., 4cm or 15cm depth of sediment that will be scraped off the bottom), the size and shape of the sediment plume, the composition and density of this sediment plume, how long the sediment contaminates the water, how far this sediment travels, what happens when the sediment settles (and which seabed life systems will be disrupted), will the chemistry of the water be affected, whether this sediment could contaminate any of the thousand-year-old slow-moving nutrient-rich deep global ocean currents[130], how mining operations plan to discard any sediment that rises to the surface in the 4.5km long ‘riser’ pipes[131], how regulators plan to monitor such sediment (industry self-regulation?), how the sediment reacts under different weather conditions given likely stormy weather for significant periods, what happens if sediment in the High Seas drifts into the National Waters of various countries and impacts deep ocean coral systems of these countries.
Incredibly, very few meaningful scientific studies have been undertaken to understand the nature of Sediment Plumes created by Seabed Mining. US university MIT has only just been commissioned to study these sediment plumes[132], and a major three-year EU research project suggest these toxic sediment clouds would be present for several months and could travel thousands of miles across the ocean through slow-moving deep ocean currents[133].
This could create the largest pollution cloud ever seen on the planet, suspended underwater for months at a time. This is at a time when the global ocean is experiencing a great de-oxygenation event[134]that was last seen during the last Mass Extinction, and ocean acidity has increased by over 30% in the past sixty years[135]. It would be incredibly risky to authorize such mining activity against an already destabilized ocean environment.
Hence it has been disingenuous for Seabed Mining companies to claim that the mining footprint of Seabed Mining operations is small, only taking into account where machines are operating and ignoring these large Sediment Plumes which would make the impact several orders of magnitude greater than any mining activity on land that the world has ever seen. At the same time, it is disingenuous and not a like-for-like comparison to show the small footprint of where the Seabed Mining machines operate, compared with the pollution and tailings of land-based open-cast mining that is displaced sediment (i.e., the very sediment that Seabed Mining operations are selectively ignoring in their impact analysis). The world needs this scientific understanding before taking any such decision that could disrupt global ocean circulation patterns with potentially catastrophic results. Particularly, when there is no clear urgency why Seabed Mining has to begin commercially in 2020.
For some of these environmental risks, there is no comparison with land-based mining in terms of how catastrophic seabed mining could be for the planet with an area equivalent to 6% of the surface of the moon[136]already licensed. This would then create the moral hazard that once commercial licensing has begun, few safeguards are in place to prevent the entire Seafloor being opened up to extraction activity in the future. This would rapidly accelerating the decline of environments that scientists barely understand - and may hold the secrets to the origins of life - with relatively few safety valves to restore these environments back again within human-timescales.
D) Pricing Sovereign and Regulatory Risk

The concept of Seabed Mining was established with the 1982 Law of the Seas, and came into operation with the International Seabed Authority in 1994[137]. This was during a very different era and value system in the midst of the Cold War. The original vision was that the International Seabed Authority should ensure the Seabed is governed for the benefit of the Common Heritage of Mankind (UN language)[138].
As scientists have discovered more about the deep ocean (and more broadly the planetary crisis), their thinking has radically altered and the current fiscal, regulatory and institutional frameworks are no longer aligned with the scientific and social consensus around Seabed Mining. This is likely to cause a period of regulatory instability and uncertainty if social, scientific and legal pressures are exerted on the ability of Seabed Mining minerals to enter the global supply chain.
Here are eight of the biggest Fiscal, Regulatory and Institutional Risks.
§ Royalty Rates: Royalty Regimes are designed to reflect the social and environmental costs of mining operations, and ensure sufficient funds are set aside for remediation. Members of the International Seabed Authority are actively debating the Royalty Regime[139] to balance the uncertain costs of ‘unknown-unknown’ environmental risks with the need to ensure equitable distribution of funds to developing countries. However, these have not yet been decided and Commercial Extraction Legislation may be voted on in the absence of agreement on the Royalty Regime.
By way of a comparison, land-based Royalty Rates are complex to set, requiring teams of economists to understand various implications, such as local job creation, international benchmarks, environmental remediation, competitiveness of ore grade. For the land-based minerals similar to those being sourced from the Seabed, there is a broad alignment around the level of Royalty Rates:
o Nickel Sulfide: the major Nickel producers pay a Royalty Regime of 15% on mineral sales[140](e.g., the very large, newly discovered High-Grade Nova-Bollinger Nickel Sulfide Mine[141]in Australia)
o Nickel Laterites: major Nickel Laterite exporter (with a similar profile to Seabed Mining), Indonesia set its Nickel Royalty Rates at 10%of sales[142]
o Cobalt: for High Grade Cobalt, the DR Congo (producer of over 68% of global Cobalt), set its Royalty Rates at 10% of sales[143]
o Copper: to reflect the higher polluting nature of open-cast mining, major Copper producer Zambia introduced a 20% Royalty Rate for open-cast mining in 2014, and 8%Royalty Rate for underground mining[144].
o Manganese: South Africa is the world’s largest Manganese producer and sets a 7% Royalty Rate[145] on sales of unrefined Manganese, and a 5% Royalty Rate on refined Manganese (to encourage greater value-add within country).
o These are all on average 500% higher than the rates that the Seabed Mining industry and the International Seabed Authority has been advocating for among members.
Representatives of the Seabed Mining Industry, certain Member States and the International Seabed Authority have been arguing for a Royalty Rate of between 2% - 4% of sales (pages 15 and 44[146]), which is one-fifth that of international norms. Four similar fiscal models have been proposed to ISA members, with China arguing that Royalty Rates should not exceed 2%[147], and the ISA has commissioned MIT to justify such rates[148]. This would effectively be a significant subsidy (80% lower than average land-based mining Royalty Rates) for minerals sourced from seabed compared with land-based Mining, who have to pay the fair share of environmental remediation costs on land. This essentially creates a lower offshore tax race to the bottom that undermines land-based Royalty Regimes put in place to compensate local communities for environmental damage. It is not clear why minerals sourced from Seabed Mining would need to receive more favorable fiscal treatment (to artificially move them to the left hand side of the industry cost curves), especially with greater environmental uncertainty and risk that would imply a higher Royalty Rate would be needed. In addition, the ISA Royalty Rate will need to reflect demands from non-seabed mining nations that proceeds from mining through royalties be equally shared with poorer countries who see the Seabed as being for the Common Heritage of all Mankind – present and future generations. All this raises questions about where minerals from Seabed Mining would truly be on the industry cost curves if they were held at the same environmental and fiscal standards as higher grade, lower polluting land-based minerals.
§ Transparency: The land-based Mining Industry has had to introduce some of the most stringent anti-corruption and transparency legislation after decades of scrutiny and high-profile cases around environmental damage[149], safety issues[150], corruption[151] and poor management[152]. This resulted in the World Bank-supported Extractive Industries Transparency Initiative[153], supported by 52 leading mining countries around the world, and overseen by an independent Board consisting of former heads of state[154] to strengthen public trust in this body. Clear operational guidelines are given on every aspect of financial transactions in mining[155], that all major mining companies and countries support and for which there are regular independent audits. Within the ISA’s Commercial Mining Legislation that is being voted on in July, the two paragraphs addressing ‘prevention of corruption,’ do not compel mining companies or countries to adhere to EITI standards (Regulation 40 in the Mining Code[156]). By not setting such an international standard (as is the norm with other pieces of international legislation), this risks a ‘race to the bottom’ of jurisdiction-shopping for companies that can secure the most favorable terms. This is particularly evident with the actions of Seabed-Mining sponsoring states such as Nauru who have had their own issues with Phosphate Mining and lack of transparency, waste and corruption over the State-backed fund earned from mining royalties that was once $1.7 billion[157] at its peak[158].
§ Sovereign Risk: As currently drafted, the ISA legislation will significantly increase sovereign risk from Seabed Mining liabilities, for countries who can least afford to take such risks, and with limited financial upside for these nations’ citizens. Seabed Mining Companies like Deep Green have secured three small island states to sponsor and underwrite their mining applications,[159]promising large royalty streams to these nations (the details of which have not been revealed for citizens to scrutinize). However, this economic analysis raises doubts whether such royalties will appear at the levels promised, given where Seabed Mining Companies claim they will be on the industry cost curve and with royalties paid to the ISA. Seabed Mining-sponsoring small island states such as Tonga, Nauru, Cook Islands, Kiribati all have GDPs of less than $500m[160], and likely even more significant debt emerging from the COVID-19 slowdown in tourism and fisheries. The cost of BP’s Deepwater Horizon offshore cleanup was over $65 billion,[161] orders of magnitude greater than these sponsoring state’s ability to fund such a cleanup operation. The ISA’s Seabed Mining Code specifies that sponsoring states are ultimately liable for any environmental damages caused by the Seabed Mining operations they sponsor (not the ISA[162]), and that companies should ensure private insurance covers this risk (without reference to what standards this entails, Regulation 36[163]). The all-important independent Article IV Inspections by the IMF to assess sovereign financial stability has not even focused on the implications of this increased sovereign risk in their recent Article IV inspections of Kiribati[164], Nauru[165], Cook Islands[166], Tonga.[167] It would be prudent for an independent IMF assessment of any sovereign risk liability from the currently drafted ISA Legislation prior to inexperienced, poorer countries rushing in to sponsor companies without full awareness of the implications on their national balance sheets.
The World Bank has already highlighted the risks and liabilities for Pacific Islands Sponsoring States (page 31[168]). This is based on a unanimous decision by a body of the International Tribunal for the Law of the Sea (ITLOS) in 2011[169], when Nauru was attempting to understand the limits of its liabilities for any environmental damage due to Seabed Mining. The Advisory Opinion given by the Seabed Disputes Chamber of the ITLOS, entitled “Responsibilities and Obligations of States Sponsoring Persons and Entities with respect to Activities in the Area,” makes it clear that smaller nations would not be shown any preferential treatments in terms of liabilities due to environmental damage (pages 55-60[170], with judgement on page 74).
§ Industry Self-Regulation: As currently drafted, the ISA Mining Legislation relies heavily on a model of industry self-regulation to decide which areas to protect, environmental standards to follow and how to monitor compliance. This creates perverse incentives to underreport environmental measures (as seen in other industries relying on such industry-self regulation approaches[171]) and significantly increases environmental risks. In the Indian Ocean, it has been left to individual contractors to decide which vent systems to protect, and which ones can be mined. These standards should have been set centrally and large environmental protection areas set aside based on independent scientific assessments rather than allowing the seabed mining industry to cherry-pick where they mine based largely on commercial considerations. In the Pacific Ocean, the ISA is heavily reliant on industry-self reporting for science and monitoring (Regulations 44-53[172]). This has resulted in weak industry oversight (the deep ocean has some of the fewest sampling datapoints upon which licensing decisions are being based[173]). Indeed, just a simple word search of the proposed ISA Legislation reveals ten times more mention of ‘Exploitation’ (201) than ‘Protection’ (24), reflecting the bias of the current legislation toward production and the Seabed Mining industry rather than safeguarding the environment for the Common Heritage of Mankind, one of the 13 original principles of the 1982 UN Law of the Sea that established the ISA, and which was originally geared more toward environmental protection, scientific discovery and ensuring benefit to all mankind (UN language), not just for a few private contractors[174].
§ Overlap with other UN Treaties: The rush to pass Seabed Mining Commercial Legislation in July 2020 does not make logical sense. 2020 was supposed to be the super-year for the environment[175]. An important Summit by the UN’s Convention on Biodiversity in December 2020 was due to set biodiversity and protection targets for the planet for next decade,[176] there is a major new UN Treaty that is in the final stages of being agreed which is focused on Biodiversity in the High Seas[177] (where Seabed Mining is licensed to take place), and UNESCO has defined 2020-2030 as the Decade of Ocean Science to truly understand the deep ocean ecosystem[178]. Due to COVID-19, these Summits have been postponed to early 2021. Given how material UN Laws and Protection Areas will be to the operations of Seabed Mining (and the fact that there is no urgency to begin Seabed Mining immediately), it would be more prudent to await the outcomes of these legislations rather than rushing through with weaker environmental Seabed Mining standards that would have to be repealed to comply with standards set in other UN Treaties, and avoiding altogether the likely complex international legal mess of overlapping jurisdictions of which environmental standards to follow that we are heading towards. These pieces of UN Biodiversity Protection legislation are material if they define particular habitats that need to be protected or better understood (e.g., hydrothermal vents, or particular nodule fields), which would be more responsible to set centrally based on independent ocean scientific advice prior to issuing mining licenses.
§ Regulatory capability: There are several significant institutional risks with the current ISA Framework that should be subject to an independent ‘Fit for Purpose’ Review.
o Resources: The ISA has an Annual Operating Budget of under $20m and less than 100 full time staff in the Secretariat, responsible for setting legislation, authorizing permits and monitoring compliance over a jurisdiction that covers 45% of the world’s surface. By comparison, South Africa – whose territory is 0.3% that of the ISA’s jurisdiction – has a budget of over $100m for programs in its Department of Mineral Resources and over 1100 full time staff[179] - ten times as many as the ISA - to oversee South Africa’s mining activities.
o Technical Competence: This has led to a series of issues within the ISA. For example, a separate body under the UN Secretary General’s Office assesses claims of Extending the Continental Shelf (CLCS of Commission on the Limits of the Continental Shelf[180]). The ISA should be ensuring that it does not issue Mining Licenses in areas that may be claimed as part of the extended continental shelf of a state. Brazil has exerted a strong claim with the CLCS over the Rio Grande Rise in the Atlantic Ocean[181], where the ISA has already issued mining licenses[182] under the ISA Mining Code (an area where it may not ultimately have jurisdiction over). In the Indian Ocean, in 2014 the ISA issued a thirty-year Exploration License to the Republic of South Korea within 200 miles of the coast of Mauritius[183]. It turns out that further assessments of the continental shelf around Mauritius reveal that this could form part of Mauritius’ extended continental shelf (an area known as the Marie Celeste Fracture Zone), which Mauritius has now submitted to the UN Secretary General[184] for recognition. This would place the ISA-issued South Korea’s Mining Concession within the territorial waters of Mauritius. This raises very serious questions about the technical ability of the ISA to be taking such decisions, and what other operational lapses this could lead to.
o Democratic oversight: The voting structure of the ISA is through a complex, three part structure involving a 167 member state ISA General Assembly, a gerrymandered five tiered and 36 member ISA Council[185], a 30 person ISA Legal and Technical Commission[186], and the requirement that all members have to physically be present in Jamaica for a vote to count[187]. This has strongly tipped the scales of the ISA Framework to strongly favor pro-Seabed Mining nations and industries who can afford to send representatives to these meetings[188] (usually fewer than half of the ISA General Assembly members eligible to vote are able to fly in).
o Conflict of interests over ISA mandate: The International Seabed Authority is acting in three capacities:
§ It is acting as a Regulator of Seabed Mining activities, including taking decisions on setting mining and environmental standards, monitoring compliance, deciding on actions and consequences of non-compliance (judge, jury and executor).
§ It is also acting as an Investment Promotion Agency to promote Seabed Mining Activities around the world[189] (e.g., through workshops[190], promotional material[191], videos[192], and investment promotion events around the world[193]).
§ Third, it is preparing a mandate to also become a Seabed Mining Operatorin its own right[194] through a policy called ‘The Enterprise[195].’ It is already engaged in discussions about a joint-venture mining operation with Poland.[196]
These are clear mandate conflicts of interest for an agency to be actively exploring becoming a Mining Company, whilst also setting favorable rules to govern its own activities. Perhaps this calls for the ISA to be broken up into three constituent bodies to more cleanly and faithfully fulfil each mandate.
o Accountability: A review of the proposed ISA Seabed Mining Legislation that is due to be voted on in July 2020 reveals that it heavily concentrates decision-making authority in the hands of a single individual executive – the ISA Secretary-General – rather than an oversight committee as is best practice in other such bodies. The current British Government-backed ISA Secretary General[197], Michael Lodge – whose four-year term is up for re-election in July – would then be unilaterally responsible for 171 executive decisions over all aspects of Seabed Mining and Environmental Protection (without clearly defined oversight of these decisions) based on the Legislation as currently drafted[198]. This is a very significant concentration of authority in the hands of one executive, given the implications of each decision.
§ State Actors: Whilst the economics and environmental implications of Seabed Mining are unlikely to be commercially favorable, not all contractors are private operators. Seabed Mining License holders from China[199], South Korea[200], Japan[201] (who have an industry alliance[202]), India[203]Brazil[204], as well as a Government Consortium from Bulgaria, Cuba, the Czech Republic, Poland, the Russian Federation and Slovakia[205], are all state-backed enterprises. Their decisions will not be informed solely by market economics, especially in terms of how R&D costs and extraction costs are accounted for. This is why strong international regulations set by the ISA is essential to govern this activity, rather than being left to market forces alone.
§ Moral Hazard and Popular protests: The ISA process to issue draft legislation is creating a moral hazard by falsely legitimizing that the UN has endorsed the Seabed Mining industry. It has resulted in countries starting to open up their national waters for Seabed Mining Activities. Already, Papua New Guinea[206], Cook Islands[207], Namibia[208], New Zealand[209], Japan[210] and Mexico[211] have started to license part of their national waters for underwater mining activities. This has led to protests in many countries (e.g., Papua New Guinea[212] and New Zealand’s indigenous populations[213]), petitions signed by over 750,000 citizens[214], a call for a Moratorium on Seabed Mining supported by 558 of 666 MPs in the European Parliament[215], High Courts overturning Government decisions on Seabed Mining concessions within National Waters[216] and a coalition of leading ocean scientists[217], major international NGOs[218] and youth organizations[219] all calling for a ten year moratorium on any commercial Seabed Mining operations. There is likely to be significant public resistance going forwards for companies, countries, traders and regulators who support Seabed Mining, that could create friction in the marketplace for corporate customers that incorporate Seabed Mining minerals into their value chains. Just as measures like the Kimberley Process on traceability of conflict diamonds were used to trace the flow of diamonds along the supply chain, it is likely that a similar measure may be needed for Seabed Mining minerals, if mining legislation is passed.
Conclusion
From this economic and resource analysis, a few important facts have been established:
§ There is no urgency or need to swiftly rush toward seabed mining (certainly not for materials to meet Paris Climate Commitments).
§ Neither the science, regulations or institutions are at a maturity level where decisions on seabed mining can be safely considered.
§ It would be prudent to consider a precautionary pause on any such Seabed Mining activity until the science (and other international environmental regulations) have sufficiently advanced to better understand the full implications of seabed mining, before humans create another activity that would destabilize earth’s already under pressure systems.
§ As the July 2020 ISA vote also coincides with the election of the new Secretary General of the ISA for a next four year term, a transparent policy statement on their position on Seabed Mining from all candidates would strengthen public faith that this is an international institution acting in the best interest of the ‘common heritage of mankind.’
COVID-19 has shown how a microscopic virus has brought the world to its knees. There is so much about the deep ocean we do not know, including the importance of the role that even the smallest microscopic seabed enzyme can play in ensuring a stable planetary system. As member states of the ISA prepare their positions on Seabed Mining and the election of the new Secretary General, it is important that they reflect on how aligned these positions are with the spirit with which the original founders of the UN Law of the Sea drafted this legislation. They were the first generation to truly discover the wonders of the deep ocean, and their intent had been the pursuit of knowledge, protection of this unique environment and ensuring the benefits reach all humankind – present and future generations. This was a habitat that had - only five years prior - revealed new possibilities for the very origins of life on this planet. With newer technologies and a decade more of ocean science, how much wiser and better informed will world leaders be before taking such a consequential decision.
[1]https://www.theatlantic.com/magazine/archive/2020/01/20000-feet-under-the-sea/603040/
[2] http://dsmobserver.com/2019/06/deepgreen-raises-150-million-as-it-advances-towards-production/
[3] https://www.spglobal.com/marketintelligence/en/news-insights/blog/mining-exploration-insights-april-2020
[4] https://www.bloomberg.com/news/articles/2020-01-14/blackrock-s-tough-on-coal-plan-skirts-around-the-biggest-miners
[5] https://www.blackrock.com/uk/individual/blackrock-client-letter
[6] https://www.isa.org.jm/deep-seabed-minerals-contractors
[7] https://www.miamiherald.com/news/nation-world/world/americas/haiti/article242447116.html
[8] https://www.greencarreports.com/news/1127660_battery-electric-or-hydrogen-fuel-cell-vw-lays-out-why-one-is-the-winner
[9] https://theconversation.com/how-do-lithium-ion-batteries-work-99164
[10] https://www.autofutures.tv/2019/04/24/battery-technology/
[11] https://www.sciencedirect.com/topics/materials-science/solid-state-battery
[12]https://www.wri.org/blog/2019/10/everything-you-need-know-about-fastest-growing-source-global-emissions-transport
[13] McKinsey Report: https://www.mckinsey.com/~/media/mckinsey/industries/automotive%20and%20assembly/our%20insights/disruptive%20trends%20that%20will%20transform%20the%20auto%20industry/auto%202030%20report%20jan%202016.ashx
[14] In the EV30@30 Scenario, EVs make up 70% of all vehicle sales in China in 2030 (42% excluding two/three-wheelers). Almost half of all vehicles sold in 2030 in Europe are EVs, 37% in Japan, more than 30% in Canada and United States, 29% in India and 22% in other countries, taken together. https://www.iea.org/reports/global-ev-outlook-2019
[15] https://qz.com/1620614/electric-car-forecasts-are-all-over-the-map/
[16] https://www.iea.org/reports/global-ev-outlook-2019. In 2030, global EV sales reach 23 million and the stock exceeds 130 million vehicles in the New Policies Scenario (excluding two/ three-wheelers). In the EV30@30 Scenario, EV sales and stock nearly double by 2030: sales reach 43 million and the stock is larger than 250 million
[17]https://www.nationalgeographic.com/news/2017/09/electric-cars-replace-gasoline-engines-2040/
[18] https://www.forbes.com/sites/jeffmcmahon/2019/11/12/even-opec-expects-electric-vehicles-to-take-over-the-roads/
[19] https://theatlas.com/charts/ryfLbE5nN
[20] https://www.mckinsey.com/~/media/mckinsey/industries/automotive%20and%20assembly/our%20insights/disruptive%20trends%20that%20will%20transform%20the%20auto%20industry/auto%202030%20report%20jan%202016.ashx
[21] https://www.bcg.com/publications/2020/drive-electric-cars-to-the-tipping-point.aspx
[22] https://about.bnef.com/electric-vehicle-outlook/
[23] https://electricautonomy.ca/2019/12/03/wealth-management-giant-acknowledges-decade-of-historic-growth-ahead-for-evs/
[24] https://www.ubs.com/content/dam/WealthManagementAmericas/documents/smart-mobility-11-march.pdf
[25] https://www.morganstanley.com/ideas/ride-sharing-electric-vehicles-carbon-emissions
[26]https://www.iea.org/news/new-cem-campaign-aims-for-goal-of-30-new-electric-vehicle-sales-by-2030
[27]https://www.mckinsey.com/~/media/mckinsey/industries/automotive%20and%20assembly/our%20insights/disruptive%20trends%20that%20will%20transform%20the%20auto%20industry/auto%202030%20report%20jan%202016.ashx
[28] https://www.chemistryworld.com/news/manganese-makeover-for-lithium-ion-batteries/3008886.article
[29] http://www.chemistryexplained.com/elements/L-P/Manganese.html
[30]https://www.forbes.com/sites/woodmackenzie/2019/07/24/can-metals-supply-keep-up-with-electric-vehicle-demand/#222b0c886c9b
[31] https://www.wired.com/story/welcome-to-the-era-of-supercharged-lithium-silicon-batteries/
[32] https://www.benchmarkminerals.com/nickel-v-cobalt-the-secret-ev-battle-for-the-lithium-ion-battery/
[33] https://ark-invest.com/research/cobalt-production
[34] https://electrek.co/2020/02/18/tesla-cheaper-chinese-lithium-iron-phosphate-batteries-report/
[35]https://www.mining.com/recycled-lithium-batteries-market-to-hit-6-billion-by-2030-report/
[36] https://www.theverge.com/2019/11/6/20951807/electric-vehicles-battery-recycling
[37] https://electrek.co/2016/11/01/breakdown-raw-materials-tesla-batteries-possible-bottleneck/
[38] https://www.geologyforinvestors.com/nickel-laterites/
[39] https://pubs.usgs.gov/info/mwni_cu/
[40] http://www.balmoralresources.com/projects/grasset/nickel-deposits-explained
[41] https://www.geologyforinvestors.com/nickel-laterites/
[42] https://en.wikipedia.org/wiki/Lateritic_nickel_ore_deposits
[43] http://users.monash.edu.au/~gmudd/files/2009-CMS-01-Nickel-Sulf-v-Lat.pdf
[44] https://www.australianmining.com.au/features/the-nickel-laterite-challenge/
[45] https://miningdataonline.com/property/1425/Nova-Mine.aspx
[46] https://www.businessnews.com.au/article/Legend-punches-through-massive-nickel-copper-sulphides-in-Fraser-Range
[47] https://www.mining-technology.com/projects/nova-nickel-copper-project-balladonia/
[48] https://www.adamasintel.com/nickel-deployed-ev-batteries-september-2019/
[49] https://www.tandfonline.com/doi/abs/10.1179/1743275812Z.00000000025
[50] https://pubs.geoscienceworld.org/books/book/1890/chapter/107059712/Exploration-Discovery-and-Geology-of-the-Ntaka
[51]https://www.northernminer.com/fast-news/garibaldi-grows-footprint-at-nickel-mountain/1003813209/
[52] https://seekingalpha.com/article/4246690-top-5-nickel-producers-and-smaller-producers-to-consider
[53] https://seekingalpha.com/article/4341343-nickel-monthly-news-for-month-of-april-2020
[54] https://www.theglobalist.com/batteries-mining-cobalt-democratic-republic-of-congo/
[55] http://www.gecoproject.org/?page=katanga-geology
[56] https://www.bloomberg.com/graphics/2018-china-cobalt/
[57] https://www.benchmarkminerals.com/glencore-closes-mutanda-mine-20-of-global-cobalt-supply-comes-offline/
[58] https://www.mining-technology.com/news/kamoto-copper-sends-350-contractors-home/
[59] https://www.bloomberg.com/news/articles/2019-08-21/china-molybdenum-says-giant-congo-copper-mine-is-losing-money
[60] https://www.reuters.com/article/us-congo-mining-cobalt/cobalt-to-be-declared-a-strategic-mineral-in-congo-idUSKCN1GQ2RX
[61] https://www.reuters.com/article/us-congo-cobalt/congo-declares-cobalt-strategic-nearly-tripling-royalty-rate-idUSKBN1O220D
[62] https://www.reuters.com/article/column-russell-cobalt-congo/column-congos-cobalt-hopes-risk-the-same-ruinous-road-as-thai-rice-russell-idUSL4N1Q90XO
[63] https://www.bloomberg.com/news/features/2018-11-16/glencore-s-misadventure-in-the-congo-threatens-its-cobalt-dreams
[64] https://www.ft.com/content/bf84900a-1764-11ea-8d73-6303645ac406
[65] https://www.amnesty.org/en/latest/news/2016/01/child-labour-behind-smart-phone-and-electric-car-batteries/
[66] https://www.amnesty.org/download/Documents/AFR6231832016ENGLISH.PDF
[67] https://www.bbc.com/news/world-africa-50812616
[68] https://www.bloomberg.com/news/articles/2020-01-30/congo-government-moves-to-monopolize-artisanal-cobalt
[69] https://www.reuters.com/article/us-cobalt-prices/large-stocks-subdued-china-demand-to-cap-cobalt-prices-idUSKBN201194
[70] https://www.dw.com/en/glencores-closure-of-congolese-cobalt-mine-could-backfire/a-50101698
[71] https://www.reuters.com/article/europe-cobalt-prices/minor-metals-glencores-plans-trigger-cobalt-price-rally-to-six-month-high-idUSL5N25T3BW
[72] https://www.benchmarkminerals.com/glencore-closes-mutanda-mine-20-of-global-cobalt-supply-comes-offline/
[73] https://www.bloomberg.com/news/articles/2019-03-04/algorithms-join-hunt-for-cobalt-backed-by-gates-bezos-and-dalio
[74] https://www.mining-technology.com/news/first-cobalt-positive-results-cobalt-refinery/
[75] https://www.nsenergybusiness.com/features/top-cobalt-producing-countries/
[76] https://www.miningmetalnews.com/20190911/1287/largest-cobalt-deposit-finland-looking-workers
[77] https://www.reuters.com/article/us-usa-minerals-china/nine-countries-join-u-s-strategic-minerals-initiative-idUSKBN1WB2VP
[78] https://www.ft.com/content/e05d8582-2aef-11e9-88a4-c32129756dd8
[79] https://www.mining.com/blackstone-minerals-convinced-bc-cobalt-project-potential-rival-moroccos-bou-azzer-cobalt-district/
[80] https://www.reuters.com/article/petropavlovsk-equity/kazakh-tycoon-sells-petropavlovsk-stake-to-focus-on-battery-minerals-idUSL8N24H3ZI
[81] https://finance.yahoo.com/news/first-cobalt-reports-49-upgrade-120000458.html
[82] https://www.proactiveinvestors.com.au/companies/news/212914/australian-mines-drills-high-grade-cobalt-and-nickel-at-sconi-212914.html
[83] https://www.autoevolution.com/pdf/news_attachements/ocean-floor-could-be-the-next-el-dorado-for-ev-battery-raw-materials-142915.pdf
[84] https://about.bnef.com/blog/behind-scenes-take-lithium-ion-battery-prices/
[85] https://www.reuters.com/article/us-tesla-china-electric-exclusive/exclusive-tesla-in-talks-to-use-catls-cobalt-free-batteries-in-china-made-cars-sources-idUSKBN20C0RP
[86]https://www.nsenergybusiness.com/features/manganese-producing-countries/
[87] https://www.dailymaverick.co.za/wp-content/uploads/BM-Ed-PwCreport-inset.pdf
[88]https://www.mineralscouncil.org.za/industry-news/publications/facts-and-figures
[89] https://www.autoevolution.com/pdf/news_attachements/ocean-floor-could-be-the-next-el-dorado-for-ev-battery-raw-materials-142915.pdf
[90]https://investingnews.com/daily/resource-investing/battery-metals-investing/manganese-investing/manganese-reserves/
[91]https://www.amm.com/Article/3910510/2020-preview-5-themes-looming-for-Mn-ore.html
[92] https://www.autoevolution.com/pdf/news_attachements/ocean-floor-could-be-the-next-el-dorado-for-ev-battery-raw-materials-142915.pdf
[93] https://www.autoevolution.com/pdf/news_attachements/ocean-floor-could-be-the-next-el-dorado-for-ev-battery-raw-materials-142915.pdf
[94]https://www.copper.org/environment/lifecycle/g_recycl.html
[95]https://www.reuters.com/article/chile-mining-copper-ev/cru-cesco-electric-cars-no-game-changer-for-copper-demand-in-short-term-idUSL8N1RA6TL
[96]https://www.reuters.com/article/us-metals-base-poll/copper-prices-seen-stifled-by-growth-fears-next-year-reuters-poll-idUSKBN1X717V
[97] https://www.reuters.com/article/chile-mining-copper-ev/cru-cesco-electric-cars-no-game-changer-for-copper-demand-in-short-term-idUSL8N1RA6TL
[98]https://www.reuters.com/article/us-metals-base-poll/copper-prices-seen-stifled-by-growth-fears-next-year-reuters-poll-idUSKBN1X717V
[99] https://www.telegraph.co.uk/finance/newsbysector/industry/mining/11621791/Mongolia-to-be-transformed-by-giant-Rio-Tinto-copper-mine.html
[100] https://www.sciencedirect.com/science/article/pii/S1674987115000924
[101]https://www.greencarcongress.com/2020/04/20200423-dg.html
[102] https://www.washingtonpost.com/politics/2019/02/21/demand-congos-cobalt-is-rise-so-is-scrutiny-mining-practices/
[103]https://www.nytimes.com/2017/04/27/world/asia/philippines-mining-environment.html
[104] https://www.oecd.org/corporate/mne/mining.htm
[105] https://www.lme.com/en-GB/About/Responsibility/Responsible-sourcing
[106] https://www.reuters.com/article/us-lme-cobalt-responsiblesourcing/london-metal-exchange-to-ban-brands-not-responsibly-sourced-by-2022-idUSKCN1RZ0YY
[107]https://www.lme.com/en-GB/About/Responsibility/Responsible-sourcing
[108]https://www.kimberleyprocess.com/en/what-kp
[109]https://www.reuters.com/article/film-diamonds-movie-retail-dc/dicaprio-film-may-hurt-diamond-demand-analysts-idUSN0449065220061204
[110] https://www.bloomberg.com/news/features/2018-11-16/glencore-s-misadventure-in-the-congo-threatens-its-cobalt-dreams
[111]https://www.reuters.com/article/congo-mining/congo-creates-state-monopoly-for-artisanal-cobalt-idUSL4N2A020N
[112]https://www.mckinsey.com/~/media/McKinsey/Business%20Functions/Sustainability/Our%20Insights/Climate%20risk%20and%20decarbonization%20What%20every%20mining%20CEO%20needs%20to%20know/Climate-risk-and-decarbonization-What-every-mining-CEO-needs-to-know.ashx
[113] https://www.renewableenergymagazine.com/panorama/renewables-becomes-the-new-normal-at-remote-20191217
[114] https://www.mining-technology.com/features/going-green-renewable-energy-projects-at-mines-around-the-world/
[115]https://www.wri.org/faqs-about-how-paris-agreement-enters-force
[116]https://dosits.org/science/movement/sofar-channel/sound-travel-in-the-sofar-channel/
[117]http://www.antarctica.gov.au/about-antarctica/environment/geology/mining
[118]https://thebarentsobserver.com/en/arctic/2019/08/us-ratifies-moratorium-fishing-high-arctic-seas
[119]https://iwc.int/commercial
[120]https://www.whoi.edu/oceanus/feature/the-discovery-of-hydrothermal-vents/
[121]https://www.downtoearth.org.in/coverage/mining/mining-at-deep-sea-46049
[122]https://www.google.com/url?sa=t&rct=j&q=&esrc=s&source=web&cd=11&ved=2ahUKEwjkp5ie0qLpAhVTKH0KHccFBx44ChAWMAB6BAgDEAE&url=https%3A%2F%2Fresearch-center.amundi.com%2Fezjscore%2Fcall%2Fezjscamundibuzz%3A%3AsfForwardFront%3A%3AparamsList%3Dservice%3DProxyGedApi%26routeId%3D_dl_MDg0NDc3YTFlMjY3Mzg3MmZhZGM2NDVlNGRiMDRmYmY&usg=AOvVaw1Uo2PqyZBj6d9vzoWKnWlK
[123] https://www.bbc.com/news/science-environment-38366118
[124] https://www.nature.com/articles/d41586-019-02242-y
[125]https://www.nature.com/articles/s41598-019-44492-w
[126]https://advances.sciencemag.org/content/6/18/eaaz5922
[127]https://www.scientificamerican.com/article/just-how-little-do-we-know-about-the-ocean-floor/
[128]https://www.forbes.com/sites/nishandegnarain/2020/03/16/will-ocean-seabed-mining-delay-the-discovery-of-potential-coronavirus-vaccines/
[129]https://www.frontiersin.org/articles/10.3389/fmars.2017.00418/full
[130]http://earthguide.ucsd.edu/virtualmuseum/climatechange1/10_5.shtml
[131]https://www.reuters.com/article/us-deep-sea-mining/belgian-fast-moving-caterpillar-in-deep-sea-copper-cobalt-race-idUSKCN1QG1B1
[132] http://news.mit.edu/2019/understanding-impact-deep-sea-mining-1206
[133] http://www.eu-midas.net/sites/default/files/downloads/MIDAS_research_highlights_low_res.pdf
[134]https://www.bbc.com/news/science-environment-50690995
[135]https://www.pmel.noaa.gov/co2/story/What+is+Ocean+Acidification%3F
[136]https://www.forbes.com/sites/nishandegnarain/2020/04/16/ten-areas-where-covid-19-responses-are-leading-to-environmental-setbacks/
[137]https://www.bbc.co.uk/news/resources/idt-sh/deep_sea_mining
[138]http://www.lse.ac.uk/iga/assets/documents/publications/2017/common-of-heritage-of-mankind-deep-sea-mining.pdf
[139]https://ran-s3.s3.amazonaws.com/isa.org.jm/s3fs-public/files/documents/mit.pdf
[140]http://www.dmp.wa.gov.au/Minerals/Royalties-1544.aspx
[141]https://www.irmau.com/site/PDF/e8e334ce-38a9-4c21-9844-ebae7dbd6ac7/NovaSiteVisitPresentation
[142]https://www.reuters.com/article/us-indonesia-nickel-tax/indonesia-nickel-ore-royalties-double-in-regulations-shake-up-idUSKBN1YE1IE
[143]https://www.reuters.com/article/us-congo-cobalt/congo-declares-cobalt-strategic-nearly-tripling-royalty-rate-idUSKBN1O220D
[144]https://www.cmi.no/publications/5730-the-rise-and-fall-of-the-mining-royalty-regime-in
[145]https://www.pwc.com/gx/en/energy-utilities-mining/publications/pdf/pwc-gx-miining-taxes-and-royalties.pdf
[146]https://ran-s3.s3.amazonaws.com/isa.org.jm/s3fs-public/documents/EN/Regs/FinTerms2014.pdf
[147]https://dsmobserver.com/2019/03/trying-to-grasp-financial-model/
[148]https://dsmobserver.com/2020/01/negotiations-continue-over-the-financial-model/
[149]https://www.theguardian.com/world/2019/jan/29/the-river-is-dying-the-vast-ecological-cost-of-brazils-mining-disasters
[150]http://www.rmmagazine.com/2014/08/01/worlds-deadliest-mine-disasters/
[151]https://www.theguardian.com/business/2019/dec/05/serious-fraud-office-investigates-glencore-over-suspected-bribery
[152]https://news.mongabay.com/2019/01/with-its-3-85b-mine-takeover-indonesia-inherits-a-13b-pollution-problem/
[153]https://eiti.org/
[154]https://eiti.org/about/board
[155]https://eiti.org/about/how-we-work
[156]https://www.isa.org.jm/document/isba25cwp1-0
[157]https://www.theguardian.com/world/2016/aug/10/a-short-history-of-nauru-australias-dumping-ground-for-refugees
[158]https://www.theguardian.com/world/2018/sep/04/corruption-incompetence-and-a-musical-naurus-riches-to-rags-tale
[159]https://www.oceannews.com/news/subsea-intervention-survey/deepgreen-acquires-tonga-offshore-mining-limited
[160]https://www.worldbank.org/en/country/smallstates/overview
[161]https://www.reuters.com/article/us-bp-deepwaterhorizon/bp-deepwater-horizon-costs-balloon-to-65-billion-idUSKBN1F50NL
[162]https://sdg.iisd.org/news/isa-adopts-strategic-plan-discusses-draft-regulations/
[163]https://www.isa.org.jm/document/isba25cwp1-0
[164]https://www.imf.org/en/Publications/CR/Issues/2019/01/24/Kiribati-2018-Article-IV-Consultation-Press-Release-Staff-Report-and-Statement-by-the-46546
[165]https://www.imf.org/en/Publications/CR/Issues/2020/01/29/Republic-of-Nauru-2019-Article-IV-Consultation-Press-Release-Staff-Report-and-Statement-by-49001
[166]https://www.imf.org/en/Publications/CR/Issues/2019/08/14/Cook-Islands-Technical-Assistance-Report-International-Financial-Services-Industry-48585
[167]https://www.imf.org/en/Publications/CR/Issues/2018/01/17/Tonga-2017-Article-IV-Consultation-Press-Release-and-the-Staff-Report-for-Tonga-45566
[168]http://pubdocs.worldbank.org/en/125321460949939983/Pacific-Possible-Deep-Sea-Mining.pdf
[169]https://www.itlos.org/cases/list-of-cases/case-no-17/
[170]https://www.itlos.org/fileadmin/itlos/documents/cases/case_no_17/17_adv_op_010211_en.pdf
[171]https://www.bbc.com/news/business-34324772
[172]https://www.isa.org.jm/document/isba25cwp1-0
[173]https://op.europa.eu/en/publication-detail/-/publication/aca8c05f-c875-11e9-9d01-01aa75ed71a1/language-en/format-PDF
[174]https://www.un.org/depts/los/convention_agreements/texts/unclos/unclos_e.pdf
[175]https://www.oecd-forum.org/users/42276-marco-lambertini/posts/57273-2020-vision-the-super-year-for-the-planet-and-ourselves
[176]https://www.cbd.int/doc/press/2019/pr-2019-09-05-cop15-en.pdf
[177]https://www.iucn.org/theme/environmental-law/our-work/oceans-and-coasts/marine-biodiversity-areas-beyond-national-jurisdiction-bbnj
[178]https://en.unesco.org/ocean-decade
[179]https://www.dmr.gov.za/resources
[180]https://www.un.org/Depts/los/clcs_new/clcs_home.htm
[181]https://dsmobserver.com/2019/10/one-of-the-most-significant-deep-sea-mining-negotiations-is-underway-at-the-commission-on-the-limits-of-the-continental-shelf/
[182]https://www.isa.org.jm/contractors/exploration-areas
[183]https://www.isa.org.jm/contractors/exploration-areas
[184]https://www.un.org/depts/los/clcs_new/submissions_files/mus36_09/MRS-ES-DOC_R1.pdf
[185]https://www.isa.org.jm/authority/council-structure-mandate
[186]https://www.isa.org.jm/authority/legal-and-technical-commission
[187]https://dsmobserver.com/2020/04/the-deep-sea-mining-community-adapts-to-the-new-normal-under-coronavirus-restrictions/
[188]http://dsmobserver.com/2019/03/the-five-most-talked-about-moments-from-the-isa-general-council-meeting/
[189]https://www.isa.org.jm/workshop/workshop-fostering-cooperation-promote-sustainable-development-africa%E2%80%99s-deep-seabed-0
[190]https://www.isa.org.jm/workshop/asean-bimstec-workshop-deep-seabed-resources-and-blue-economy
[191]http://www.deepseaminingoutofourdepth.org/why-the-rush/
[192]http://www.deepseaminingoutofourdepth.org/wp-content/uploads/Why-the-Rush.pdf
[193]https://www.isa.org.jm/news/secretary-general-michael-lodge-makes-deep-sea-mining-presentation-hamburg-business-club
[194]http://dsmobserver.com/2019/03/the-five-most-talked-about-moments-from-the-isa-general-council-meeting/
[195]https://www.isa.org.jm/news/1st-part-25th-annual-session-international-seabed-authority-isa-highlights-thursday-28-february
[196]https://www.isa.org.jm/document/isba24c12
[197]http://www.jamaicaobserver.com/news/seabed-authority-votes-for-secretary-general-today_67795
[198]https://www.isa.org.jm/document/isba25cwp1-0
[199]https://chinadialogueocean.net/9771-china-deep-sea-mining-contract/
[200]http://www.kiost.ac.kr/eng/sub02_01.do
[201]https://ran-s3.s3.amazonaws.com/isa.org.jm/s3fs-public/files/documents/4-japan.pdf
[202]http://www.dord.co.jp/english/about/index.html
[203]https://www.thehindu.com/sci-tech/science/why-is-india-pulled-to-deep-sea-mining/article28809029.ece
[206]https://www.theguardian.com/world/2019/sep/16/collapse-of-png-deep-sea-mining-venture-sparks-calls-for-moratorium
[207]https://www.theguardian.com/world/2019/oct/20/cook-islands-manager-of-worlds-biggest-marine-park-says-she-lost-job-for-backing-sea-mining-moratorium
[208]https://www.mining-technology.com/features/marine-mining-lessons-from-namibia/
[209]https://www.newsroom.co.nz/2019/10/08/848216/nz-support-for-seabed-mining-on-offer-in-pacific#
[210]https://www.bbc.com/future/article/20181221-japans-grand-plans-to-mine-deap-sea-vents
[211]https://www.mining-technology.com/news/mexicos-federal-court-rules-favour-odyssey-marines-mining-permit/
[212]https://www.civicus.org/index.php/media-resources/news/interviews/4275-papua-new-guinea-if-we-allow-seabed-mining-then-we-may-just-call-for-the-end-of-humanity
[213]https://www.rnz.co.nz/news/national/413512/environmental-group-lauds-victory-for-the-oceans-after-mining-company-loses-appeal
[214]https://secure.avaaz.org/campaign/en/deep_sea_mining_loc/
[215]https://www.newsdeeply.com/oceans/articles/2018/02/01/european-parliament-calls-for-a-moratorium-on-deep-sea-mining
[216]https://www.rnz.co.nz/news/national/365127/high-court-overturns-south-taranaki-seabed-mining-application
[217]https://www.fauna-flora.org/news/ffi-calls-for-moratorium-on-deep-sea-mining
[218]https://www.conservation.org/press-releases/2020/01/20/conservation-international-calls-for-a-minimum-ten-year-moratorium-on-deep-sea-mining
ExO Insight Newsletter
Join the newsletter to receive the latest updates in your inbox.